Cellular and Circuit Mechanisms of Action Selection
Deep Brain Stimulation (DBS) and its Mechanisms in Movement Disorders
Function of the Healthy and Diseased Basal Ganglia in Cognitive Control
Eye Movement Control Systems in Movement Disorders
Cellular and Circuit Mechanisms of Action Selection
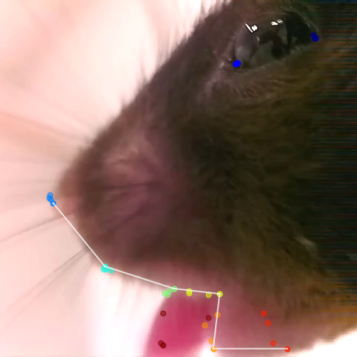
Freeze frame of PNKD mouse experiencing orofacial dyskinesia following caffeine injection.
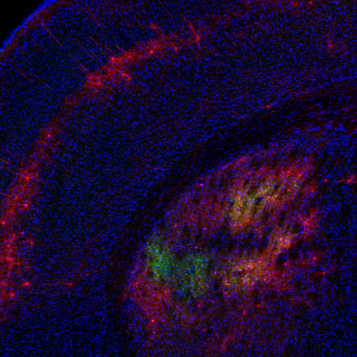
Coronal brain slice showing rabies tracing of TRAPed striatal neurons.
The basal ganglia are a group of interconnected subcortical structures that have key roles in the selection of appropriate actions. In the movement domain, activity within the basal ganglia are hypothesized to facilitate desired movements and inhibit competing (undesired) motor programs. Many neurological disorders affecting the basal ganglia are characterized by impaired voluntary movement, including bradykinesia, hypokinesia and tremor (as are seen in Parkinson’s Disease), or by involuntary movements, including dyskinesias (involuntary and erratic movements of the face, arms, legs or trunk) and dystonia (involuntary muscle contractions that often lead to twisting movements). In the lab, we are interested in understanding the underlying neural mechanisms of these movement disorders.
Parkinson’s Disease and levodopa-induced dyskinesia
The progressive loss of midbrain dopamine neurons is thought to underlie the debilitating motor deficits associated with Parkinson’s Disease (PD). For more than 50 years, the gold-standard therapy has been the administration of the dopamine precursor levodopa, which is thought to restore dopamine release and thereby restore many aspects of movement. However, long-term use of levodopa often leads to the development of involuntary movements known as levodopa-induced dyskinesia (LID). While the movement abnormalities in both PD and LID are dopamine-dependent, how chronic changes in dopamine alter cellular and circuit function are still poorly understood.
To better understand the mechanisms underlying PD and LID, one of our projects is focused on the striatum, a structure critical for motor function that receives dense dopaminergic inputs and gives rise to the direct and indirect pathways of the basal ganglia. Prevailing theories have speculated that an imbalance in activity between the direct and indirect pathway medium spiny neurons (dMSNs and iMSNs, respectively), are thought to contribute to the loss of normal voluntary movement in these disorders. Using optogenetically-labeled recordings (“photo-tagging”) of dMSNs and iMSNs in parkinsonian mice, we have shown that dopamine loss and levodopa administration have differential effects on the firing of striatal neurons. To investigate how striatal activity changes with repeated, long-term use of levodopa, we are currently performing longitudinal recordings of calcium signals in dMSNs and iMSNs. During LID in particular, we have shown that levodopa produces heterogeneous effects on dMSN activity, with a distinct subpopulation of dMSNs preferentially activated during LID. Using an activity-dependent mouse line, FosTRAP, to capture and manipulate cells activated during LID, we have also found that TRAPed striatal neurons are both necessary and sufficient for producing dyskinesia. Interestingly, TRAPed striatal neurons represent a subpopulation of predominantly dMSNs. Using the FosTRAP mouse line to gain access to dyskinesia-related striatal neurons, we are currently investigating the cellular and synaptic mechanisms underlying their role in LID. This project is utilizing cell type-specific retrograde tracing techniques, as well as ex vivo whole-cell recordings, combined with pharmacology and optogenetics.
Another project aims to understand how aberrant basal ganglia activity may drive thalamocortical dynamics in PD and LID. The basal ganglia process cortical information and send feedback to the cortex via the motor nuclei of the thalamus, which is likely to contribute to the initiation and execution of voluntary movements. In PD, the thalamus and motor cortices show alterations in movement-related firing activity and changes in local field potential (LFP) oscillations that correlate with PD motor symptoms. In fact, PD therapies such as levodopa administration or DBS regularizes cortical activity, supporting that aberrant activity from the basal ganglia might be propagated to the cortex via the thalamus contributing to impaired movement in PD. To better understand the activity and synaptic changes in the basal ganglia recipient zone of the motor thalamus in PD and LID, we are combining slice electrophysiology with in vivo electrophysiological recordings and fiber photometry in the 6-OHDA mouse model of PD.
Paroxysomal nonkinesigenic dyskinesia
In some cases, involuntary movements are related to a specific lesion or loss of cells in the brain (as in Huntington’s Disease), but in other cases the brain looks normal on MRI. This observation implies that abnormal movements may be driven by abnormal patterns of brain activity or connectivity, rather than a frank loss of cells. The fact that neuromodulatory treatments, like Deep Brain Stimulation (DBS) can be effective in treatment of dystonia supports this idea. But what cells and what patterns of activity drive dyskinetic movements? Many theorize aberrant activity may arise in movement control circuitry like the basal ganglia and cerebellum. To investigate the connections and patterns of activity that generate involuntary movements, we use a variety of mouse models.
One major project in the lab focuses on the PNKD (paroxysmal nonkinesigenic dyskinesia) mouse model, developed by our UCSF colleague Louis Ptacek. This model is based on a human autosomal dominant disorder of the same name, in which patients experience attacks of involuntary movements lasting hours, after drinking alcohol or coffee, or experiencing extreme stress. Mice with the same gene mutations found in patients have normal-looking brains, but also have these attacks, triggered by administration of alcohol or caffeine. PNKD mice allow us to investigate the neural correlates of dyskinetic attacks, using in vivo single-unit recordings in freely moving animals. We recently published work using optogenetically labeled single-unit recordings, as well as DREADDs (designer receptors exclusively activated by designer drugs) to test longstanding theories in the field that speculate that dyskinesia may be facilitated by an imbalance in direct and indirect pathway striatal activity. Currently, we are using this same model to explore mechanisms in downstream basal ganglia nuclei, specifically in the context of dystonic attacks after the administration of alcohol.
Tardive Dyskinesia
Another project focuses on tardive dyskinesia (TD), a movement disorder induced by specific medications that block dopamine transmission, such as the antipsychotic drug haloperidol. TD is characterized by involuntary and twisting movements of the jaw, lips and tongue. Mice exposed to chronic haloperidol also develop spontaneous, involuntary abnormal chewing activity (called vacuous chewing movements or VCMs) which resemble the signs of TD in humans. We are currently combining in vivo bulk imaging of neural activity and/or dopamine release (using fiber photometry with GCaMP or dLight), to understand the neural correlates of orofacial movements, and how they change from healthy to pathological states.
Deep Brain Stimulation (DBS) and its Mechanisms in Movement Disorders
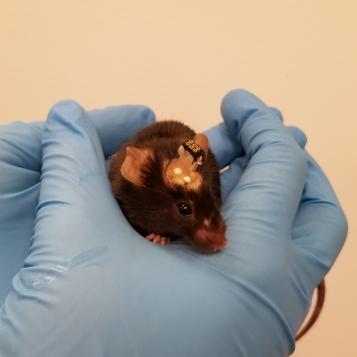
Parkinsonian mouse with DBS and fiber photometry implants.
For the last 25 years, many people with Parkinson’s Disease have been implanted with an electrical stimulator in the subthalamic nucleus (STN), which sends pulses directly into the brain. In more recent years, the same technique (though often in different parts of the brain) has been used to treat a wide variety of other disorders, such as Dystonia, Obsessive-Compulsive Disorder (OCD) and Tourette syndrome. While deep brain stimulation (DBS) therapy is quite effective in treating core movement symptoms of Parkinson’s Disease, it remains unclear exactly how it works and when it should be used. Without this knowledge, it is challenging to optimize DBS for current patients, and to predict which patients might benefit from DBS in the future. This gap in knowledge extends to our understanding some aspects of the underlying neural circuits, including the basal ganglia, which regulate movement in both in healthy and disease states.
One major impediment to filling this gap has been a lack of models allowing for artifact-free, cell-specific interrogation of basal ganglia circuitry during DBS. With this problem in mind, we developed a model of STN DBS in parkinsonian mice. A mouse model, combined with the mouse genetic toolbox and many of the cutting-edge optical tools available for use in mice, can allow cell type-specific recording of neural activity, as well as manipulations of neural activity, to explore how DBS relieves parkinsonism. In this model, we can use fluorescent calcium indicators (which aren’t susceptible to the electrical artifacts of traditional electrophysiology) to record from specific regions and cell types of the mouse brain during electrical stimulation. Using this model, we are working to precisely capture changes in different brain structures in response to stimulation. We hope this work will put us on the path to understanding and perhaps improving DBS for PD and dystonia as well as implementing it for other neuropsychiatric diseases. Additionally, we have worked to characterize the behavioral response of parkinsonian mice to a wide variety of DBS parameters, resulting in a metric that can be used to predict stimulation efficacy. Currently, we are conducting causal experiments using the knowledge we’ve gained through bulk calcium recordings and hope to soon incorporate single-cell calcium recordings as well.
Function of the Healthy and Diseased Basal Ganglia in Cognitive Control
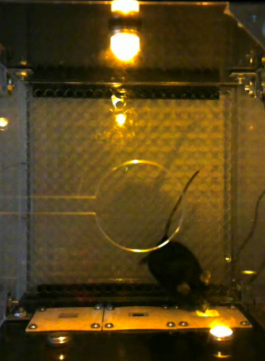
A mouse conducting operant behavior training.
Parkinson’s disease (PD) should not be considered merely a movement disorder. PD patients also commonly suffer from a broad range of non-motor symptoms, including anxiety, depression, disrupted sleep patterns, and impaired cognition. Cognitive dysfunction is one of the most disabling symptoms of PD. While PD medications and deep brain stimulation (DBS) are all effective in mitigating motor symptoms, several studies have demonstrated that they have poor, and even negative, cognitive effects on PD patients. Of these negative effects, impulse control disorder (ICD) is saliently observed in about 15% of PD patients receiving dopamine agonists. Despite the serious symptoms of ICD, including binge eating, gambling, and hypersexuality, little is known about why dopamine agonists increase the risk of ICD and what the underlying neural mechanism is.
To better understand ICD, there is a need for comprehensive studies in rodents, aimed at establishing a behavioral model to reproduce ICDs and understanding the neural encoding mechanism underlying impulsivity in Parkinson's disease. Currently, we are combining mouse operant training with in-vivo electrophysiology and optogenetics to examine striatal activity in both healthy mice and ICD model mice during different behavioral tasks, which may render the potential mechanisms underlying ICD. We are also hoping to use in-vitro electrophysiology to explore the abnormal connectivity between the striatum and upstream regions in an ICD model, which could cause the activity changes in-vivo.
Eye Movement Control Systems in Movement Disorders
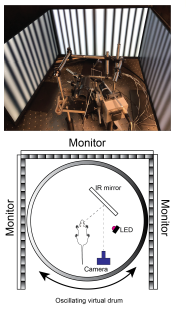
Picture of head-fixed setup (top) and schematic of experimental set up (bottom).
Progressive supranuclear palsy (PSP) is a complex neurodegenerative disorder affecting at least 20,000 people in the U.S. Clinically, PSP presents with similar hypokinetic movements seen in patients with Parkinson’s disease (PD) and is typically misdiagnosed as PD. Unique to PSP, is the early presentation gaze palsy, i.e, slowed saccadic eye movements, and horizontal square wave jerks. Neuropathologically, postmortem analysis of PSP brain tissue revealed the presence of insoluble aggregates of the microtubule associated protein Tau in several motor control regions, with the earliest affected areas being basal ganglia nuclei such as the subthalamic nucleus (STN), Globus pallidus (GP), striatum, and substantia nigra pars reticulata (SNr). While PSP bear similar clinical presentation to PD, dopamine replacement therapies used to treat PD are largely ineffective in typical PSP. The circuit mechanisms driving the motoric symptoms of PSP are currently unknown, largely due to lack of animal models of disease. Using a Tau transgenic mouse to model PSP we assess:
1. The validity of Tau transgenic mouse to recapitulate the gross locomotor and neuropathological aspects of PSP
2. Whether Tau transgenic mice have impaired saccadic eye movements
3. The involvement of basal ganglia output on saccade parameters in healthy and Tau transgenic mice.